Quasi-periodic pulsations (QPPs) are specified as intensity modulations in the flare electro-magnetic radiation as a function of time. These modulations have been found to have characteristic periodicities that range from < < 1 s as much as several minutes. QPPs were very first connected with the impulsive phase of flares and observed in the difficult X-ray (HXR) and radio wavebands (Parks & & Winkler 1969). They have more recently been observed within the thermal element of flares and have actually been recognized to persist into the decay phase (Dennis et al. 2017). Thus studies that explore signatures of QPPs across the whole electromagnetic spectrum are very important to help us develop a photo of their underlying chauffeur. Recent research studies have identified that a lower limit of ~ 46 % of the X-class flares in the last solar cycle included QPPs (Hayes et al. 2020). Regardless of the ubiquity of QPPs in flares, the underlying system that produces them stays unknown. Several models have actually been proposed as explanations for the existence of QPPs, which are normally classified as oscillatory or self-oscillatory processes (McLaughlin et al. 2018). Oscillatory procedures incorporate mechanisms in which magnetohydrodynamic (MHD) oscillations or waves modulate the electromagnetic emission, or promote magnetic reconnection on a time-dependent basis. These processes permit seismological possibilities of using QPPs to probe outstanding and solar flare websites to extract properties such as the electron density, electromagnetic field, and flare loop lengths. Self-oscillatory procedures are translated as a symptom of time-dependent, intermittent magnetic reconnection. These processes enable for the presence of broad-band QPPs and if discovered to be happening, would supply new insight into the nature of energy release in flares. Observations & & Results In this work, we investigate a GOES M3.7 flare that happened on 2015 November 4. The flare exhibited noticable QPPs throughout a broad-band of wavelengths. We recognize QPPs in the X-ray, low-frequency radio, and extreme ultraviolet (EUV) wavebands. We figure out that these QPPs are an effect of intermittent particle velocity, likely due to "bursty" magnetic reconnection. At the energy release site, accelerated electrons precipitate toward the chromosphere to produce the X-ray and EUV pulsations. The electrons accelerated towards interplanetary area escape along open magnetic field lines, leading to low-frequency radio pulses in the type of type III radio bursts. Current studies have actually determined that a lower limit of ~ 46 % of the X-class flares in the last solar cycle consisted of QPPs (Hayes et al. 2020). In spite of the universality of QPPs in flares, the underlying system that produces them stays unidentified. We analyze the QPPs recognized in this flare in terms of pulsed electron acceleration caused by time-dependent intermittent reconnection. In Figure 4 we reveal a cartoon scenario of the flare site to highlight how the QPP sources are related to the magnetic field setup. Following each burst of electron velocity, those that get away up along the open magnetic field lines result in the type III QPPs, and those that take a trip along closed lines precipitate in the chromosphere to trigger the QPPs we observe in hard X-ray and EUV. Figure 1-- (a) reveals the dynamic spectrum of the radio emission consisting of a sequence of type III radio bursts and Figure 1( b) shows the EUV, soft X-ray (SXR), and HXR light curves in which we recognize seven pulsations. The EUV light curves were extracted from the QPP source region determined in Figure 2. The EUV, hxr, and sxr QPPs were discovered to have the exact same duration of ~ 2 minutes, suggesting a common progenitor. Lines drawn from the peaks of pulses 1 and 7 from the 171 Å curve indicate the time delay required for the electron beams to reach this height from the flare site. We have related QPPs observed in the SXR, HXR, EUV, and low frequency radio programs. Locating the QPP Source Figure 2-- Spatial analysis of the QPPs. Left: an AIA 171 Å image with RHESSI 35-70 keV image contours overlaid. The light curves on the best program the HXRs (full Sun) with the EUV emission drawn out from 2 of the kernels marked in the image, revealing K1 to be the main source area. RHESSI imaging was employed to figure out where the non-thermal HXRs stemmed. It was discovered that there were three HXR sources on the map which are labelled as K1, k3, and k2. These sources are displayed in red overlaid on the 171 Å SDO/AIA image in Figure 2. To identify the area within the flare site primarily producing the QPPs, we incorporated the emission from each EUV image over each area of the active region using different kernel sizes, created light curves for each of these kernels for the period of the flare, and compared the profiles of the time series to that of the HXR emission. The emission from K1 produced the most prominent QPPs and was discovered to yield a periodicity that best matched the HXR pulsations. A natural escape route Figure 3-- PFSS extrapolation revealing the geometry of the electromagnetic field lines of the flaring region overlaid on the AIA 171 Å image. The QPP source area, K1, is connected with open magnetic field lines, recognized in the prospective field source surface (PFSS) extrapolation displayed in Figure 3. This magnetic field geometry enables a mechanism for the escape of the electrons accountable for producing the radio emission. Conclusions Figure 4-- Cartoon of the flaring region highlighting the likely system through which we observe the episodic particle velocity leading to QPPs in EUV, sxr, hxr, and radio. We interpret the QPPs recognized in this flare in regards to pulsed electron acceleration triggered by time-dependent intermittent reconnection. In Figure 4 we show an animation scenario of the flare website to show how the QPP sources belong to the magnetic field setup. Following each burst of electron acceleration, those that leave upward along the open electromagnetic field lines result in the type III QPPs, and those that travel along closed lines precipitate in the chromosphere to trigger the QPPs we observe in hard X-ray and EUV. This work supplies new evidence that time-dependent or oscillatory reconnection can naturally generate QPPs, supplying an explanation for their existence across the entire spatial variety of flaring emission. It shines light onto the nature of energy release in flares, provides insight into how QPPs be can localised to specific areas of flare sites, which QPPs can manifest over vast ranges through several emission system. Future work to examine the conditions and details needed for the triggering of magnetic reconnection in this bursty style is needed.
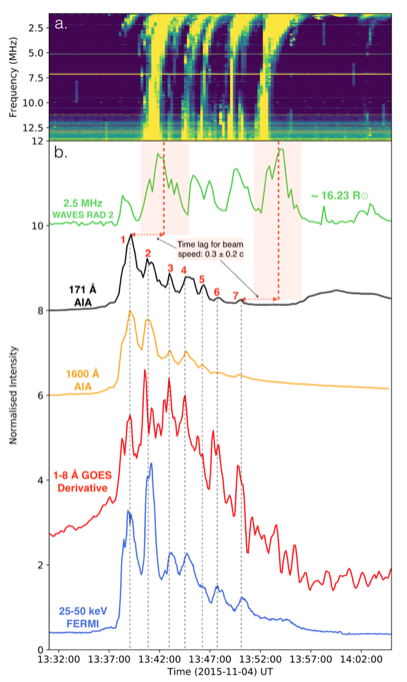