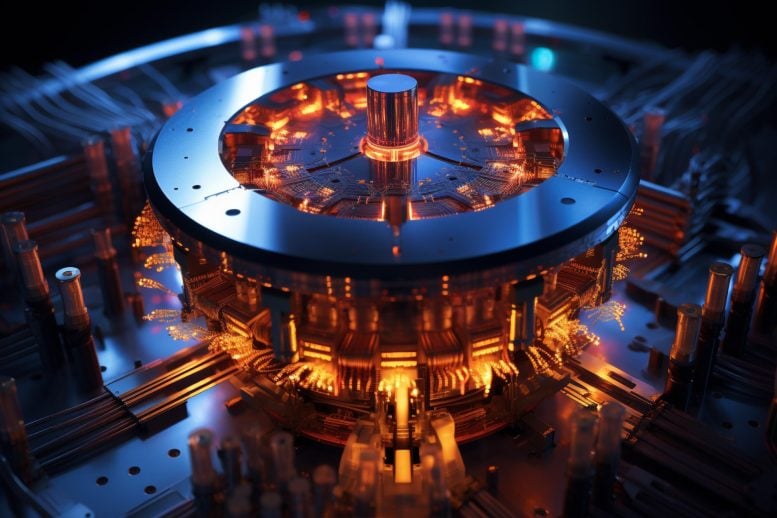
Researchers at Caltech have developed a novel method for measuring the error rates of quantum computers using classical computers, without the need for full simulations. This breakthrough helps overcome the challenges posed by the inherent errors in quantum systems and could accelerate the development of more accurate quantum computing technologies. (Artist’s concept.) Credit: SciTechDaily.com
A new method employs classical computers to verify the accuracy of complex quantum systems.
Quantum computers hold the promise of surpassing classical computers in solving complex problems across various domains such as computer science, medicine, business, chemistry, and physics. However, these advanced machines have yet to reach their full potential, as they currently struggle with inherent errors. Researchers are diligently working to minimize these flaws and enhance the performance of quantum computers.
One way to study these errors is to use classical computers to simulate the quantum systems and verify their accuracy. The only catch is that as quantum machines become increasingly complex, running simulations of them on traditional computers would take years or longer.
Now, Caltech researchers have invented a new method by which classical computers can measure the error rates of quantum machines without having to fully simulate them. The team describes the method in a paper in the journal Nature.
“In a perfect world, we want to reduce these errors. That’s the dream of our field,” says Adam Shaw, lead author of the study and a graduate student who works in the laboratory of Manuel Endres, professor of physics at Caltech. “But in the meantime, we need to better understand the errors facing our system, so we can work to mitigate them. That motivated us to come up with a new approach for estimating the success of our system.”
Quantum Simulators and Entanglement
In the new study, the team performed experiments using a type of simple quantum computer known as a quantum simulator. Quantum simulators are more limited in scope than current rudimentary quantum computers and are tailored for specific tasks. The group’s simulator is made up of individually controlled Rydberg atoms—atoms in highly excited states—which they manipulate using lasers.
One key feature of the simulator, and of all quantum computers, is entanglement—a phenomenon in which certain atoms become connected to each other without actually touching. When quantum computers work on a problem, entanglement is naturally built up in the system, invisibly connecting the atoms. Last year, Endres, Shaw, and colleagues revealed that as entanglement grows, those connections spread out in a chaotic or random fashion, meaning that small perturbations lead to big changes in the same way that a butterfly’s flapping wings could theoretically affect global weather patterns.
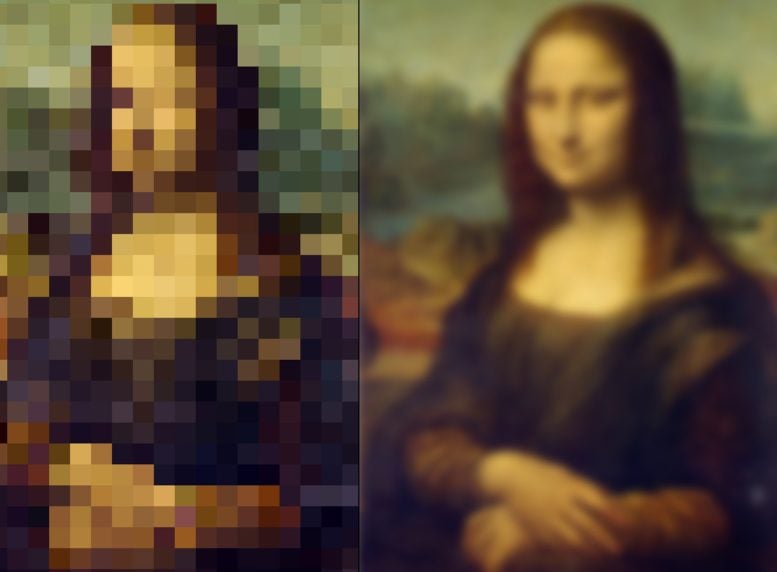
Classical computers are not capable of fully simulating the work of quantum systems, especially those with more than 30 qubits. As a hypothetical example, if a quantum computer were to create the Mona Lisa, it could create many of the details but is prone to errors, hence the blurry appearance of the painting on the right in this artist’s concept. A classical computer could not create the details as well as the quantum computer but could roughly approximate the task of the quantum computer, hence the pixelation of the image on the left. To get around this limitation and better simulate quantum systems, researchers used a method to change the amount of “pixelation” in a classical computer simulation and then extrapolate from those results to estimate the “blurriness” of quantum systems. Credit: Caltech
Limits of Classical Simulations
This increasing complexity is believed to be what gives quantum computers the power to solve certain types of problems much faster than classical computers, such as those in cryptography in which large numbers must be quickly factored.
But once the machines reach a certain number of connected atoms, or qubits, they can no longer be simulated using classical computers. “When you get past 30 qubits, things get crazy,” Shaw says. “The more qubits and entanglement you have, the more complex the calculations are.”
The quantum simulator in the new study has 60 qubits, which Shaw says puts it in a regime that is impossible to simulate exactly. “It becomes a catch-22. We want to study a regime that is hard for classical computers to work in, but still rely on those classical computers to tell if our quantum simulator is correct.” To meet the challenge, Shaw and colleagues took a new approach, running classical computer simulations that allow for different amounts of entanglement. Shaw likens this to painting with brushes of different sizes.
“Let’s say our quantum computer is painting the Mona Lisa as an analogy,” he says. “The quantum computer can paint very efficiently and, in theory, perfectly, but it makes errors that smear out the paint in parts of the painting. It’s like the quantum computer has shaky hands. To quantify these errors, we want our classical computer to simulate what the quantum computer has done, but our Mona Lisa would be too complex for it. It’s as if the classical computers only have giant brushes or rollers and can’t capture the finer details.
“Instead, we have many classical computers paint the same thing with progressively finer and finer brushes, and then we squint our eyes and estimate what it would have looked like if they were perfect. Then we use that to compare against the quantum computer and estimate its errors. With many cross-checks, we were able to show this ‘squinting’ is mathematically sound and gives the answer quite accurately.”
The researchers estimated that their 60-qubit quantum simulator operates with an error rate of 91 percent (or an accuracy rate of 9 percent). That may sound low, but it is, in fact, relatively high for the state of the field. For reference, the 2019 Google experiment, in which the team claimed their quantum computer outperformed classical computers, had an accuracy of 0.3 percent (though it was a different type of system than the one in this study).
Shaw says: “We now have a benchmark for analyzing the errors in quantum computing systems. That means that as we make improvements to the hardware, we can measure how well the improvements worked. Plus, with this new benchmark, we can also measure how much entanglement is involved in a quantum simulation, another metric of its success.”
Reference: “Benchmarking highly entangled states on a 60-atom analogue quantum simulator” by Adam L. Shaw, Zhuo Chen, Joonhee Choi, Daniel K. Mark, Pascal Scholl, Ran Finkelstein, Andreas Elben, Soonwon Choi and Manuel Endres, 20 March 2024, Nature.
DOI: 10.1038/s41586-024-07173-x
The research was funded by the National Science Foundation (partially via Caltech’s Institute for Quantum Information and Matter, or IQIM), the Defense Advanced Research Projects Agency (DARPA), the Army Research Office, the U.S. Department of Energy’s Quantum Systems Accelerator, the Troesh postdoctoral fellowship, the German National Academy of Sciences Leopoldina, and Caltech’s Walter Burke Institute for Theoretical Physics.