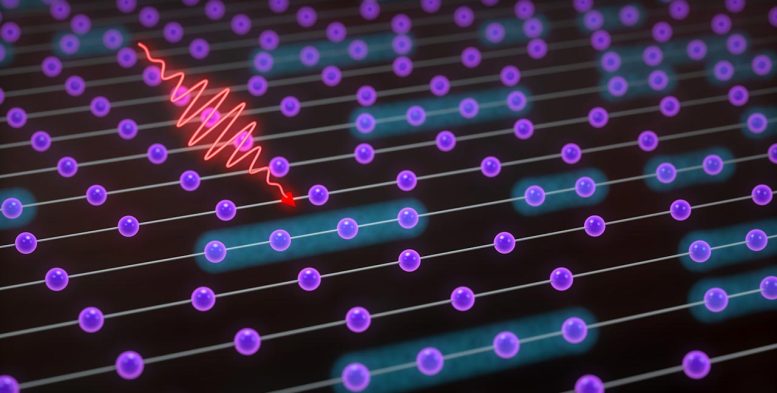
Photoinduced bipolaron-to-polaron formations distorting a quasi-1D lattice of atoms play a major role in the formation of the pseudogap. Credit: Steven Burrows/Murnane and Kapteyn Groups
Innovative use of timed laser pulses by JILA and CU Boulder researchers provides new insights into quantum material behavior, aiding in the exploration of superconductivity mechanisms.
To engineer materials with unique properties, like superconductivity, scientists dive into the quantum interactions between electrons and vibrational particles called phonons. When electrons and phonons strongly interact, they behave as “quasi” particles, not single isolated particles. These interactions occur on extremely short timescales: electrons interact with each other in femtoseconds (10-15 seconds) or even faster, while phonons respond more slowly, within hundreds of femtoseconds, since the heavier atoms move more slowly than electrons.
To investigate these interactions, scientists often change a material’s temperature, pressure, or chemical composition and measure its electrical properties to learn about the interactions. However, materials that host different interactions can exhibit very similar properties, making it challenging to pinpoint the exact nature of these interactions.
To overcome this issue, JILA graduate student Yingchao Zhang, working with JILA Fellows Henry Kapteyn and Margaret Murnane and University of Colorado Boulder physics professor Rahul Nandkishore, utilized a powerful new method to precisely identify phonon interactions within quantum materials, the results of which were published in the journal Nano Letters. Using ultraprecise, timed laser pulses, and extreme ultraviolet pulses, they measured the response times and saw precisely how the electrons and phonons interacted. This method paves the way for better control and manipulation of quantum materials.
Unraveling One-Dimensional Quantum Materials
In this new study, the researchers compared how the electrons in two different materials responded after they were gently perturbed by light: (TaSe4)2I and Rb0.3MoO3, also known as rubidium blue bronze. These materials are one-dimensional (1D) because, as shown in the corresponding figure, they have strong bonds along one direction, with weaker bonds in a perpendicular direction. This forces the electrons and phonons to interact strongly with each other, making the properties of these materials very dependent on quantum phenomena.
Historically, both materials were thought to have a small insulating gap produced by the coupling between electrons and phonons, called a polaron. This insulating gap can create problems when trying to understand the quantum interactions within polarons, as it becomes challenging to stimulate any interactions within the material.
However, a recent study from Stanford University, done in parallel with this experimental work, suggests that the insulating gap in some materials could be produced instead by polarons interacting to produce bipolarons (or polaron pairs). Because small bipolarons share similar properties to bosons, a fundamental particle, some experts have theorized that bipolarons could create a type of Bose-Einstein-Condensate (BEC), which could be a mechanism for superconductivity in the material.
The JILA and CU Boulder researchers pointed out that their experiment could be naturally explained in this bipolaron scenario, indicating that the (TaSe4)2I material is a bipolaron insulator. “It is a great example of how theory and experiment, working together, can lead to new insights,” explained Nandkishore.
Beyond Material Relaxation Times
To do this, the team used ultrafast laser pulses to gently excite several electrons within the two materials. Then, an ultrafast extreme UV pulse, at a wavelength ten times shorter than visible light, was used to see exactly where the electrons were excited in energy and location. By tracking the energy and location of the excited electrons, the researchers could see the signatures of bipolarons melting into single polarons in (TaSe4)2I.
Beyond understanding what interactions give rise to the insulation gap, the researchers also observed different relaxation times in their two materials. Relaxation time, or the time needed for a material to recover from stress, heat, or light, varies based on the quantum interactions within the material.
In (TaSe4)2I, the atoms in the lattice need to rearrange as bipolarons melt into single polarons. This process takes around 250 femtoseconds, followed by a slow relaxation to the bipolaron ground state within 1500 femtoseconds, as shown in the corresponding figure.
“The ability to see the location of excited electrons, and measure their relaxation times, provide[s] new insights into the microscopic interactions in these materials, inaccessible to traditional experimental techniques,” Nandkishore added.
In contrast, the electrons in Rb0.3MoO3 responded and relaxed ten times faster in response to light (in approximately 60 femtoseconds), clearly showing that interactions between electrons must be responsible for the insulating gap in that 1D material. This faster relaxation time seems consistent with a different physics theory known as the Luttinger-liquid theory.
In a Luttinger liquid, the electrons move more like a crowd at a concert instead of moving like individuals. They interact strongly with each other and form a kind of collective behavior. This collective behavior makes the liquid act like an insulator, refusing to conduct an electrical current.
This new method, demonstrated by the JILA and CU Boulder researchers, can also be used to reveal the nature of the quantum quasiparticle interactions in other materials, such as superconductors and 2D materials.
“We are excited that by being able to precisely probe the interactions between electrons, phonons, and spins in materials on their fundamental time scales, we can uncover why these materials have the properties they have and also learn how to manipulate them,” Murnane stated.
Reference: “Bipolaronic Nature of the Pseudogap in Quasi-One-Dimensional (TaSe4)2I Revealed via Weak Photoexcitation” by Yingchao Zhang, Chaitanya Murthy, Tika R. Kafle, Wenjing You, Xun Shi, Lujin Min, Huaiyu Hugo Wang, Na Li, Venkatraman Gopalan, Zhiqiang Mao, Kai Rossnagel, Lexian Yang, Henry Kapteyn, Rahul Nandkishore and Margaret Murnane, 8 September 2023, Nano Letters.
DOI: 10.1021/acs.nanolett.3c01078